Diodes play a key role in electronic circuits by allowing electrical current to flow in one direction but blocking it in the opposite direction. They are widely used in various applications, such as power supply circuits, radio receivers, computers, and electric vehicles. There are different types of diodes, each with its own characteristics, advantages, and limitations. In this article, we will explore the different types of diodes, their applications, and the factors that affect their performance. By understanding these nuances, you can choose the right diode for your specific application and ensure reliable operation.
Various Types of Diodes
Here is a list of various types of diodes:
- Rectifier Diode
- Zener Diode
- Light Emitting Diode (LED)
- Schottky Diode
- Tunnel Diode
- Laser Diode
- Photodiode
- Varactor Diode
- PIN Diode
- Gunn Diode
- Avalanche Diode
- Backward Diode
- Transient Voltage Suppression (TVS) Diode
- Gold Doped Diode
- Constant Current Diode
- Step Recovery Diode
- Peltier or Thermal Diode
- Vacuum Diode
Rectifier Diode
A rectifier diode is a type of electronic component that is used to convert alternating current (AC) into direct current (DC). It is a semiconductor device that allows current to flow in only one direction, effectively blocking the flow of current in the opposite direction. This property makes rectifier diodes ideal for use in power supplies, where they are used to convert the AC voltage from the mains into a DC voltage that can be used to power electronic devices.
Rectifier diodes are available in a variety of different types, including silicon and germanium diodes. Silicon diodes are the most commonly used type of rectifier diode, as they are more efficient and have a higher voltage rating than germanium diodes. They are also more durable and have a longer lifespan.
Rectifier diodes are typically rated based on their maximum forward current and reverse voltage. The maximum forward current is the maximum amount of current that the diode can handle when it is conducting current in the forward direction. The reverse voltage is the maximum voltage that the diode can withstand when it is blocking current in the reverse direction.
One important consideration when using rectifier diodes is their voltage drop. When a diode is conducting current in the forward direction, it will have a voltage drop across it. This voltage drop can range from a few tenths of a volt to several volts, depending on the type of diode and the amount of current flowing through it. This voltage drop can be a significant factor in the design of power supplies, as it can affect the efficiency and output voltage of the supply.
In summary, rectifier diodes are an essential component in electronic devices that require DC power. They are used to convert AC voltage into DC voltage and are available in a variety of different types and ratings. When using rectifier diodes, it is important to consider their voltage drop and other specifications to ensure that they are suitable for the intended application.
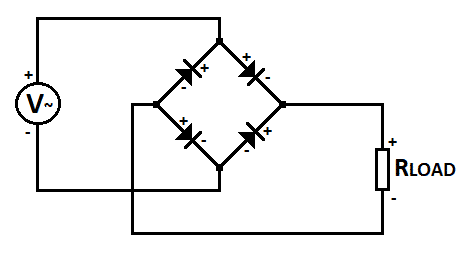
Zener Diode
A Zener diode is a type of semiconductor diode that is designed to operate in the reverse breakdown region of its voltage-current characteristic curve. Unlike regular diodes, which are designed to conduct current in the forward direction and block current in the reverse direction, Zener diodes are designed to conduct current in the reverse direction when a certain voltage, known as the Zener voltage, is reached.
Zener diodes are commonly used as voltage regulators in electronic circuits. When a Zener diode is connected in parallel with a load, it can maintain a constant voltage across the load, even if the input voltage varies. This is because the Zener diode will conduct current in the reverse direction when the voltage across it exceeds the Zener voltage, effectively regulating the voltage across the load.
Zener diodes are available in a range of different voltage ratings, from a few volts to several hundred volts. They are typically rated based on their Zener voltage, maximum power dissipation, and maximum current rating. The Zener voltage is the voltage at which the diode begins to conduct in the reverse direction. The maximum power dissipation is the maximum amount of power that the diode can safely dissipate without overheating. The maximum current rating is the maximum amount of current that the diode can safely handle.
One important consideration when using Zener diodes is their temperature coefficient. The Zener voltage of a diode can vary with temperature, which can affect the accuracy of the voltage regulation. To address this issue, Zener diodes are often designed with a temperature compensation circuit, which adjusts the voltage across the diode to compensate for temperature changes.
In summary, Zener diodes are a type of semiconductor diode that is designed to operate in the reverse breakdown region of their voltage-current characteristic curve. They are commonly used as voltage regulators in electronic circuits and are available in a range of different voltage ratings and specifications. When using Zener diodes, it is important to consider their voltage rating, maximum power dissipation, maximum current rating, and temperature coefficient to ensure that they are suitable for the intended application.
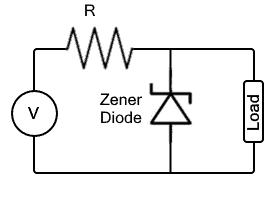
Light Emitting Diode (LED)
A Light light-emitting diode (LED) is a semiconductor device that emits light when an electric current is passed through it. LEDs are widely used in a variety of applications, including lighting, displays, and indicators, due to their energy efficiency, long lifespan, and low heat output.
LEDs are made up of a semiconductor material, typically a combination of gallium, arsenic, and phosphorus, that is doped with impurities to create a p-n junction. When a voltage is applied to the p-n junction, electrons and holes recombine, releasing energy in the form of photons, which produce light.
One of the key advantages of LEDs is their energy efficiency. Unlike traditional incandescent bulbs, which produce light by heating a filament, LEDs produce light directly from the movement of electrons in a semiconductor material. This means that they use significantly less energy to produce the same amount of light, making them more environmentally friendly and cost-effective.
Another advantage of LEDs is their long lifespan. LEDs can last up to 25 times longer than traditional incandescent bulbs, which means that they require less frequent replacement and maintenance. This makes them ideal for use in applications where access is difficult or where frequent replacement is impractical.
LEDs are also available in a wide range of colors, including red, green, blue, and white. This makes them ideal for use in displays and indicators, where different colors are required to convey information.
In recent years, LED technology has advanced significantly, with the development of high-power LEDs that can produce a significant amount of light from a small package. This has led to the widespread adoption of LEDs in lighting applications, where they are used to produce bright, energy-efficient light for a variety of indoor and outdoor applications.
In summary, Light light-emitting diodes (LEDs) are semiconductor devices that emit light when an electric current is passed through them. They are energy-efficient, long-lasting, and available in a wide range of colors, making them ideal for use in a variety of applications, including lighting, displays, and indicators. As LED technology continues to advance, we can expect to see even more innovative applications of this versatile technology in the future.
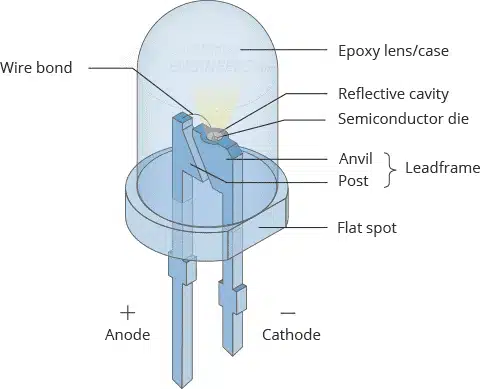
Schottky Diode
A Schottky diode is a type of electronic component that is widely used in various applications, such as power supplies, voltage regulators, and signal processing circuits. It is named after its inventor, Walter H. Schottky, a German physicist who first described its operation in 1938.
A Schottky diode is a semiconductor device that consists of a metal-semiconductor junction, where a metal contact is placed on a lightly doped semiconductor material, such as silicon or gallium arsenide. The metal contact, usually made of platinum, tungsten, or aluminum, forms a barrier with the semiconductor material, which allows current to flow in one direction only.
The main advantage of a Schottky diode over a conventional p-n junction diode is its low forward voltage drop, typically around 0.3-0.5 volts, which results in less power dissipation and higher efficiency. This is because the metal-semiconductor junction has a smaller depletion region and a faster switching speed than the p-n junction, which reduces the voltage drop and the switching losses.
Another advantage of a Schottky diode is its high-frequency response, which makes it suitable for applications that require fast switching or high-speed rectification, such as RF detectors, mixers, and modulators. The Schottky diode also has a low reverse recovery time, which means that it can switch off quickly and avoid reverse current flow.
However, a Schottky diode has some limitations, such as its low reverse voltage rating, typically around 50-200 volts, which makes it unsuitable for high-voltage applications. It also has a higher leakage current than a p-n junction diode, which can cause noise and instability in some circuits.
In summary, a Schottky diode is a useful and versatile electronic component that offers low forward voltage drop, high-frequency response, and fast switching speed. Its unique properties make it a popular choice for many applications in electronics and telecommunications.
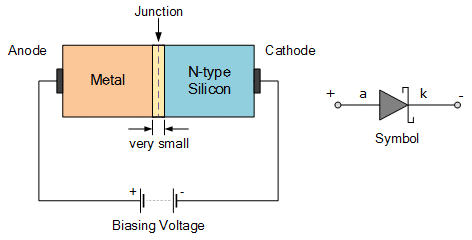
Tunnel Diode
A tunnel diode is a type of semiconductor device that exhibits a negative resistance region in its current-voltage characteristic, which allows it to operate as a high-speed switch, oscillator, or amplifier. It was first invented by Leo Esaki, a Japanese physicist, in 1957, who received the Nobel Prize in Physics in 1973 for his work on tunneling phenomena.
A tunnel diode is made of a heavily doped p-n junction, where the doping concentration is so high that it creates a thin depletion region that allows electrons to tunnel through it. This tunneling effect occurs when the energy of the electrons in the conduction band of the n-type material is lower than the energy of the holes in the valence band of the p-type material, which creates a negative resistance region in the current-voltage curve.
The main advantage of a tunnel diode over other types of diodes is its high-speed operation, which can reach frequencies of up to several gigahertz. This is because the tunneling effect occurs very quickly, without the need for charge carriers to diffuse through the depletion region, which reduces the transit time and the switching delay.
Another advantage of a tunnel diode is its low power consumption, which makes it suitable for low voltage and low current applications, such as microwave detectors, mixers, and oscillators. The tunnel diode also has a high temperature stability, which allows it to operate at high temperatures without significant degradation in performance.
However, a tunnel diode has some limitations, such as its narrow operating range, which limits its use to specific applications that require high-speed and low-power operation. It also has a low forward voltage drop, which can cause thermal instability and self-heating in some circuits.
In summary, a tunnel diode is a unique and specialized semiconductor device that offers high-speed and low-power operation, thanks to its negative resistance region and tunneling effect. Its properties make it a valuable component in many applications that require high-frequency and high-performance operation, especially in the field of telecommunications and microwave engineering.
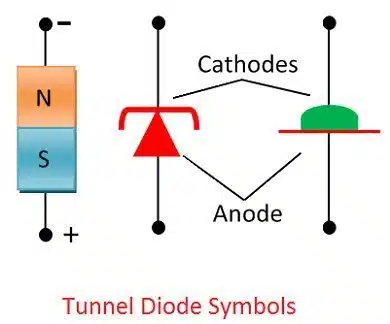
Laser Diode
A laser diode is a type of semiconductor device that emits coherent and monochromatic light through the process of stimulated emission. It is widely used in various applications, such as optical communication, data storage, medical treatment, and industrial processing. The laser diode was first invented by Robert N. Hall, a physicist at General Electric, in 1962.
A laser diode is made of a p-n junction, where the p-type and n-type materials are sandwiched between two mirrors, which form a resonant cavity that amplifies the light. When a forward bias voltage is applied to the diode, electrons, and holes are injected into the active region, where they recombine and emit photons through the process of stimulated emission.
The main advantage of a laser diode over other types of light sources is its high efficiency, compact size, and low power consumption. A laser diode can convert electrical energy into light with an efficiency of up to 70%, which makes it more energy-efficient than incandescent bulbs or fluorescent lamps. A laser diode is also small and lightweight, which makes it suitable for portable and space-constrained applications. Additionally, a laser diode can operate at low currents and voltages, which reduces power consumption and extends the battery life.
Another advantage of a laser diode is its high coherence and monochromaticity, which means that the emitted light has a narrow spectral width and a well-defined phase relationship. This property makes a laser diode suitable for applications that require precise and stable light sources, such as optical communication, spectroscopy, and interferometry.
However, a laser diode has some limitations, such as its sensitivity to temperature and current fluctuations, which can affect its performance and reliability. A laser diode also has a limited lifetime, which can be affected by factors such as aging, degradation, and external stresses.
In summary, a laser diode is a powerful and versatile semiconductor device that offers high efficiency, coherence, and monochromaticity. Its unique properties make it a valuable component in many applications that require precise and stable light sources, especially in the field of optics and photonics.
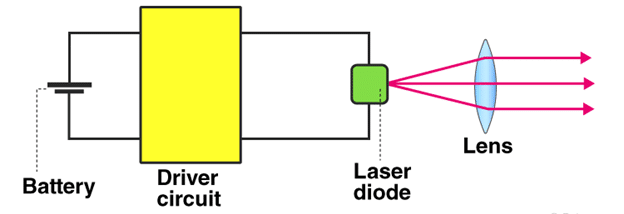
Photodiode
A photodiode is a type of semiconductor device that converts light energy into electrical energy through the process of photoelectric effect. It is widely used in various applications, such as optical communication, sensing, imaging, and detection. The photodiode was first invented by Julius Elster and Hans Geitel, German physicists, in 1905.
A photodiode is made of a p-n junction, where the p-type and n-type materials are exposed to light through a transparent window. When photons of light are absorbed by the semiconductor material, they create electron-hole pairs, which generate a photocurrent that flows through the diode. The magnitude of the photocurrent is proportional to the intensity of the incident light and the sensitivity of the photodiode.
The main advantage of a photodiode over other types of light sensors is its high sensitivity, speed, and linearity. A photodiode can detect very low levels of light, down to a few photons, which makes it suitable for applications that require high sensitivity, such as optical communication and spectroscopy. A photodiode can also respond very quickly to changes in light intensity, with response times of nanoseconds or less, which makes it suitable for applications that require high-speed detection, such as imaging and sensing. Additionally, a photodiode has a linear response to light intensity, which means that the photocurrent is proportional to the incident light, which makes it suitable for applications that require accurate and precise measurements.
Another advantage of a photodiode is its low noise and dark current, which means that it can detect very weak signals without being affected by internal noise or background radiation. A photodiode also has a wide spectral range, which means that it can detect light in a broad range of wavelengths, from ultraviolet to infrared.
However, a photodiode has some limitations, such as its sensitivity to temperature and bias voltage, which can affect its performance and stability. A photodiode also has a limited dynamic range, which means that it can saturate or clip at high light intensities.
In summary, a photodiode is a powerful and versatile semiconductor device that offers high sensitivity, speed, and linearity. Its unique properties make it a valuable component in many applications that require light detection and measurement, especially in the field of optics and photonics.
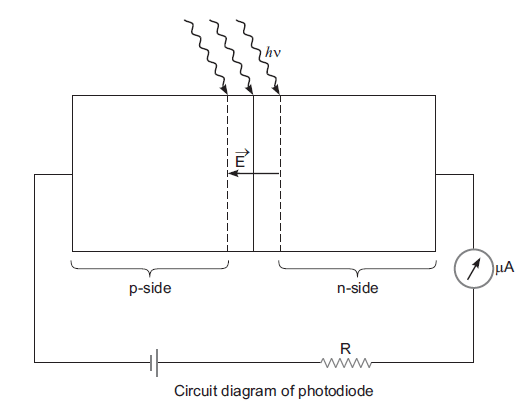
Varactor Diode
A varactor diode, also known as a varicap diode, is a type of semiconductor device that exhibits a variable capacitance when a reverse bias voltage is applied to it. It is widely used in various applications, such as voltage-controlled oscillators, frequency synthesizers, and phase-locked loops. The varactor diode was first invented by William Shockley, a physicist at Bell Labs, in 1952.
A varactor diode is made of a p-n junction, where the p-type and n-type materials are doped to create a depletion region that acts as a capacitor. When a reverse bias voltage is applied to the diode, the depletion region widens, which reduces the capacitance of the diode. The magnitude of the capacitance is proportional to the voltage applied to the diode and the doping concentration of the semiconductor material.
The main advantage of a varactor diode over other types of capacitors is its tunability and linearity. A varactor diode can vary its capacitance over a wide range of values, from a few picofarads to several hundred picofarads, which makes it suitable for applications that require voltage-controlled tuning, such as frequency modulation and demodulation. A varactor diode also has a linear response to the applied voltage, which means that the capacitance changes proportionally to the voltage, which makes it suitable for applications that require accurate and precise tuning.
Another advantage of a varactor diode is its low noise and distortion, which means that it can provide high-quality signals without introducing unwanted noise or distortion. A varactor diode also has a low power consumption, which makes it suitable for battery-powered applications.
However, a varactor diode has some limitations, such as its sensitivity to temperature and aging, which can affect its performance and stability. A varactor diode also has a limited voltage range, which means that it can be damaged or destroyed if the reverse bias voltage exceeds its breakdown voltage.
In summary, a varactor diode is a powerful and versatile semiconductor device that offers tunability, linearity, and low noise. Its unique properties make it a valuable component in many applications that require voltage-controlled tuning and frequency modulation, especially in the field of telecommunications and wireless communication.
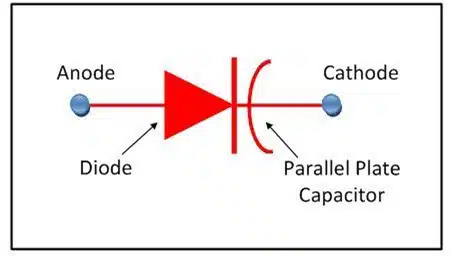
PIN Diode
A PIN diode is a type of semiconductor device that has a p-type region, an intrinsic region, and an n-type region, which form a structure similar to a p-n junction diode. It is widely used in various applications, such as RF switches, attenuators, and photodetectors. The PIN diode was first invented by William Shockley, a physicist at Bell Labs, in 1950.
A PIN diode is made of a heavily doped p-type material, a lightly doped intrinsic material, and a heavily doped n-type material, which forms a depletion region that acts as a resistor. When a forward bias voltage is applied to the diode, the depletion region narrows, which reduces the resistance of the diode. When a reverse bias voltage is applied to the diode, the depletion region widens, which increases the resistance of the diode.
The main advantage of a PIN diode over other types of diodes is its high sensitivity and low noise. A PIN diode can detect very weak signals, down to a few nanowatts, which makes it suitable for applications that require high sensitivity, such as photodetection and RF signal processing. A PIN diode also has low noise and distortion, which means that it can provide high-quality signals without introducing unwanted noise or distortion.
Another advantage of a PIN diode is its high speed and low power consumption. A PIN diode can switch on and off very quickly, with response times of nanoseconds or less, which makes it suitable for applications that require high-speed switching, such as RF switches and attenuators. A PIN diode also has a low power consumption, which makes it suitable for battery-powered applications.
However, a PIN diode has some limitations, such as its sensitivity to temperature and bias voltage, which can affect its performance and stability. A PIN diode also has a limited dynamic range, which means that it can saturate or clip at high signal levels.
In summary, a PIN diode is a powerful and versatile semiconductor device that offers high sensitivity, low noise, and high speed. Its unique properties make it a valuable component in many applications that require signal detection and processing, especially in the field of telecommunications and wireless communication.
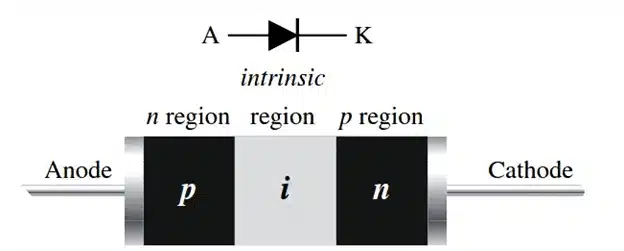
Gunn Diode
A Gunn diode is a type of semiconductor device that exhibits a negative differential resistance region in its current-voltage characteristic, which allows it to operate as a high-frequency oscillator. It is widely used in various applications, such as microwave generators, amplifiers, and detectors. The Gunn diode was first invented by J. B. Gunn, an American physicist, in 1963.
A Gunn diode is made of a single n-type material, such as gallium arsenide, which has a high electron mobility and a low ionization energy. When a forward bias voltage is applied to the diode, the electric field in the material becomes high enough to cause the electrons to move faster than the average drift velocity, which creates a negative differential resistance region in the current-voltage curve. This region allows the diode to generate microwave oscillations at frequencies of up to several gigahertz.
The main advantage of a Gunn diode over other types of oscillators is its simplicity, compactness, and low cost. A Gunn diode does not require any resonant circuit or external components to generate microwave oscillations, which makes it suitable for applications that require low-power and low-cost solutions, such as radar systems and microwave communication. A Gunn diode is also small and lightweight, which makes it suitable for portable and space-constrained applications.
Another advantage of a Gunn diode is its high-frequency response and low phase noise. A Gunn diode can generate microwave oscillations with frequencies of up to several gigahertz, which makes it suitable for applications that require high-frequency operation, such as satellite communication and wireless networks. A Gunn diode also has a low phase noise, which means that it can provide stable and accurate signals without introducing unwanted noise or distortion.
However, a Gunn diode has some limitations, such as its sensitivity to temperature and bias voltage, which can affect its performance and stability. A Gunn diode also has a limited output power, which means that it may require amplification or modulation for some applications.
In summary, a Gunn diode is a powerful and versatile semiconductor device that offers simplicity, compactness, and high-frequency operation. Its unique properties make it a valuable component in many applications that require microwave oscillation and signal generation, especially in the field of telecommunications and microwave engineering.
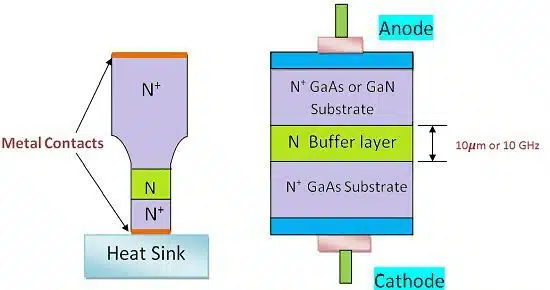
Avalanche Diode
An avalanche diode is a type of semiconductor device that exhibits a phenomenon called avalanche breakdown, which allows it to operate as a high-voltage regulator or protector. It is widely used in various applications, such as power supplies, surge protectors, and voltage references. The avalanche diode was first invented by Leo Esaki, a Japanese physicist, in 1957.
An avalanche diode is made of a p-n junction, where the p-type and n-type materials are doped to create a high electric field that can cause the electrons to gain enough energy to ionize the atoms in the material. When the voltage across the diode exceeds a certain threshold, called the breakdown voltage, the ionization process becomes self-sustaining, which creates a large current flow through the diode. This process is called avalanche breakdown, and it can occur in both the reverse and forward bias regions of the diode.
The main advantage of an avalanche diode over other types of diodes is its high voltage handling capability and low noise. An avalanche diode can withstand high voltages, up to several kilovolts, without being damaged or destroyed, which makes it suitable for applications that require high-voltage regulation or protection, such as power supplies and surge protectors. An avalanche diode also has low noise and distortion, which means that it can provide high-quality signals without introducing unwanted noise or distortion.
Another advantage of an avalanche diode is its fast response time and low capacitance. An avalanche diode can respond very quickly to changes in voltage, with response times of nanoseconds or less, which makes it suitable for applications that require high-speed switching or regulation, such as pulse generators and voltage references. An avalanche diode also has a low capacitance, which means that it can handle high-frequency signals without introducing unwanted capacitance or delay.
However, an avalanche diode has some limitations, such as its sensitivity to temperature and bias voltage, which can affect its performance and stability. An avalanche diode also has a limited dynamic range, which means that it can saturate or clip at high signal levels.
In summary, an avalanche diode is a powerful and versatile semiconductor device that offers high voltage handling capability, low noise, and fast response time. Its unique properties make it a valuable component in many applications that require high-voltage regulation or protection, especially in the field of power electronics and high-voltage engineering.
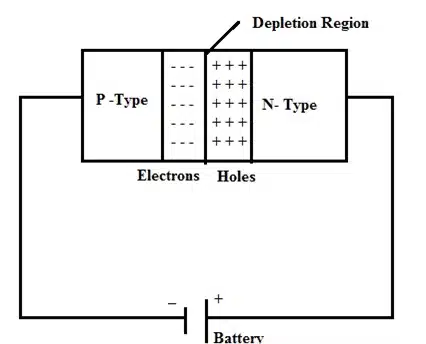
Backward Diode
A backward diode is a type of semiconductor device that exhibits a negative differential resistance region in its current-voltage characteristic, which allows it to operate as a high-frequency oscillator or detector. It is also known as a back diode or a barrier injection transit-time diode. The backward diode was first invented by Leo Esaki, a Japanese physicist, in 1957.
A backward diode is made of a p-n junction, where the p-type and n-type materials are doped to create a high electric field that can cause the electrons to tunnel through the depletion region and inject into the p-type material. When a forward bias voltage is applied to the diode, the electrons are injected into the p-type material, where they undergo a process called transit-time oscillation, which creates a negative differential resistance region in the current-voltage curve. This region allows the diode to generate microwave oscillations at frequencies of up to several gigahertz.
The main advantage of a backward diode over other types of oscillators is its high efficiency, low noise, and low power consumption. A backward diode can generate microwave oscillations with high efficiency, up to 50%, which makes it suitable for applications that require low-power and high-efficiency solutions, such as wireless communication and radar systems. A backward diode also has a low noise and distortion, which means that it can provide high-quality signals without introducing unwanted noise or distortion.
Another advantage of a backward diode is its high frequency response and low phase noise. A backward diode can generate microwave oscillations with frequencies of up to several gigahertz, which makes it suitable for applications that require high-frequency operation, such as satellite communication and wireless networks. A backward diode also has a low phase noise, which means that it can provide stable and accurate signals without introducing unwanted noise or distortion.
However, a backward diode has some limitations, such as its sensitivity to temperature and bias voltage, which can affect its performance and stability. A backward diode also has a limited output power, which means that it may require amplification or modulation for some applications.
In summary, a backward diode is a powerful and versatile semiconductor device that offers high efficiency, low noise, and high-frequency operation. Its unique properties make it a valuable component in many applications that require microwave oscillation and signal generation, especially in the field of telecommunications and microwave engineering.
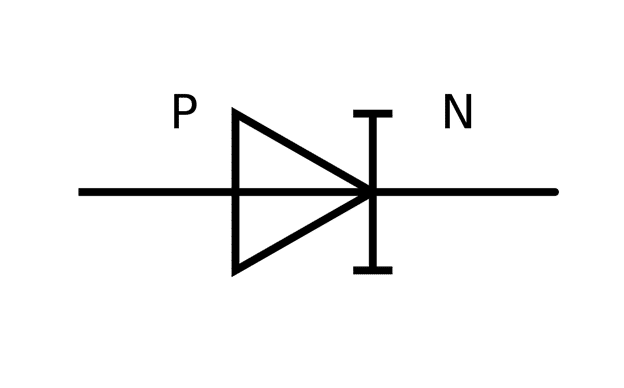
Transient Voltage Suppression (TVS) Diode
A transient voltage suppression (TVS) diode is a type of semiconductor device that is designed to protect electronic circuits from transient voltage spikes and surges, such as electrostatic discharge (ESD), lightning strikes, and power surges. It is also known as a surge protection diode or a suppressor diode. The TVS diode was first invented by General Electric in the 1970s.
A TVS diode is made of a p-n junction, where the p-type and n-type materials are doped to create a high electric field that can absorb and dissipate the energy of transient voltage spikes. When a transient voltage spike occurs, the TVS diode conducts the excess current and voltage away from the protected circuit, which prevents damage or destruction of the circuit components.
The main advantage of a TVS diode over other types of surge protection devices is its fast response time and low clamping voltage. A TVS diode can respond very quickly to transient voltage spikes, with response times of nanoseconds or less, which makes it suitable for applications that require high-speed protection, such as data communication and power electronics. A TVS diode also has a low clamping voltage, which means that it can limit the voltage across the protected circuit to a safe level, without introducing unwanted resistance or delay.
Another advantage of a TVS diode is its low capacitance and low leakage current. A TVS diode has a low capacitance, which means that it can handle high-frequency signals without introducing unwanted capacitance or delay. A TVS diode also has a low leakage current, which means that it can protect the circuit without consuming excessive power or introducing unwanted noise.
However, a TVS diode has some limitations, such as its sensitivity to temperature and current, which can affect its performance and stability. A TVS diode also has a limited energy handling capability, which means that it may require additional protection devices for high-energy transient events.
In summary, a TVS diode is a powerful and versatile semiconductor device that offers fast response time, low clamping voltage, and low capacitance. Its unique properties make it a valuable component in many applications that require transient voltage protection and surge suppression, especially in the field of power electronics and data communication.
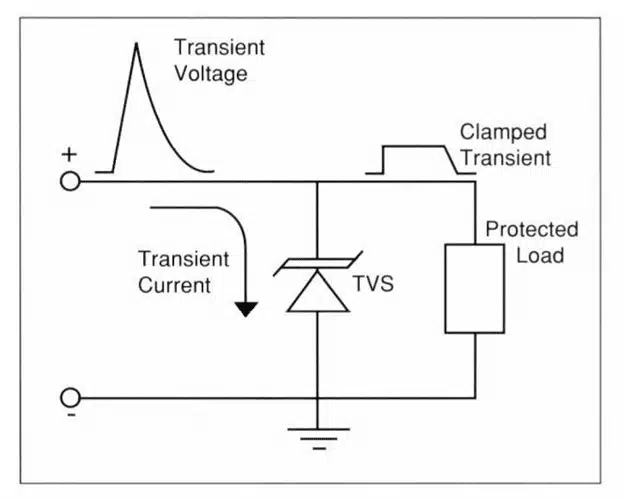
Gold Doped Diode
A gold-doped diode is a type of semiconductor device that is doped with gold atoms to create a Schottky barrier with a low barrier height and a high ideality factor. It is also known as a gold-doped Schottky diode or a gold-doped barrier diode. The gold-doped diode was first invented by M. S. Shur, a physicist at Rensselaer Polytechnic Institute, in 1980.
A gold-doped diode is made of a metal-semiconductor junction, where the metal contact is made of gold and the semiconductor material is doped with gold atoms. The gold atoms create a Schottky barrier with a low barrier height, which allows the diode to conduct current at low voltages and low temperatures. The gold-doped diode also has a high ideality factor, which means that it has a high degree of rectification and low leakage current.
The main advantage of a gold-doped diode over other types of diodes is its low voltage operation and high sensitivity. A gold-doped diode can operate at low voltages, down to a few millivolts, which makes it suitable for applications that require low-power and low-voltage operation, such as sensors and detectors. A gold-doped diode also has a high sensitivity, which means that it can detect very weak signals, down to a few nanowatts.
Another advantage of a gold-doped diode is its high frequency response and low noise. A gold-doped diode can respond very quickly to changes in voltage and current, with response times of nanoseconds or less, which makes it suitable for applications that require high-speed switching or detection, such as microwave detectors and mixers. A gold-doped diode also has a low noise and distortion, which means that it can provide high-quality signals without introducing unwanted noise or distortion.
However, a gold-doped diode has some limitations, such as its sensitivity to temperature and aging, which can affect its performance and stability. A gold-doped diode also has a limited reverse voltage rating, which means that it may require additional protection devices for high-voltage applications.
In summary, a gold-doped diode is a powerful and versatile semiconductor device that offers low-voltage operation, high sensitivity, and high-frequency response. Its unique properties make it a valuable component in many applications that require low-power and low-voltage operation, especially in the field of sensors, detectors, and microwave engineering.
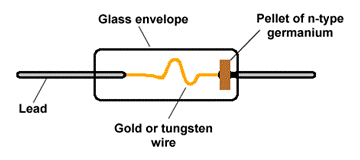
Constant Current Diode
A constant current diode, also known as a current-limiting diode or a current-regulating diode, is a type of semiconductor device that is designed to provide a constant current flow through a circuit, regardless of changes in voltage or load resistance. It is widely used in various applications, such as LED drivers, battery chargers, and voltage regulators.
A constant current diode is made of a p-n junction, where the p-type and n-type materials are doped to create a high electric field that can regulate the current flow through the diode. When a forward bias voltage is applied to the diode, the current flow through the diode is limited to a constant value, which is determined by the doping concentration and the physical dimensions of the diode.
The main advantage of a constant current diode over other types of current regulators is its simplicity, compactness, and low cost. A constant current diode does not require any external components or feedback circuits to regulate the current flow, which makes it suitable for applications that require low-power and low-cost solutions, such as LED lighting and battery-powered devices. A constant current diode is also small and lightweight, which makes it suitable for portable and space-constrained applications.
Another advantage of a constant current diode is its high efficiency and low noise. A constant current diode can regulate the current flow with high efficiency, up to 90%, which means that it can minimize the power loss and heat dissipation in the circuit. A constant current diode also has a low noise and distortion, which means that it can provide high-quality signals without introducing unwanted noise or distortion.
However, a constant current diode has some limitations, such as its sensitivity to temperature and bias voltage, which can affect its performance and stability. A constant current diode also has a limited current range, which means that it may require additional current regulation or protection devices for high-current applications.
In summary, a constant current diode is a powerful and versatile semiconductor device that offers constant current regulation, simplicity, and high efficiency. Its unique properties make it a valuable component in many applications that require current regulation and control, especially in the field of LED lighting, battery-powered devices, and power electronics.
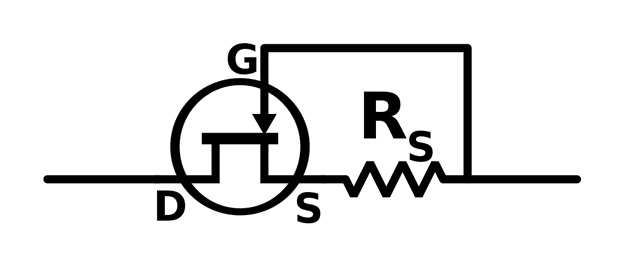
Step Recovery Diode
A step recovery diode is a type of semiconductor device that exhibits a fast-switching time and a high frequency response, which allows it to operate as a pulse generator or frequency multiplier. It is also known as a charge storage diode or a snap-off diode. The step recovery diode was first invented by H. Schumacher, a German physicist, in 1963.
A step recovery diode is made of a p-n junction, where the p-type and n-type materials are doped to create a high electric field that can store and release charge carriers. When a forward bias voltage is applied to the diode, the charge carriers are injected into the depletion region, where they are stored and accumulated. When the bias voltage is switched off, the stored charge carriers are released and flow through the diode, which creates a fast switching pulse with a high frequency content.
The main advantage of a step recovery diode over other types of pulse generators is its high frequency response and low distortion. A step recovery diode can generate fast switching pulses with rise times of picoseconds or less, which makes it suitable for applications that require high-speed switching or pulse shaping, such as radar systems and pulse generators. A step recovery diode also has a low distortion, which means that it can provide high-quality signals without introducing unwanted noise or distortion.
Another advantage of a step recovery diode is its high efficiency and low power consumption. A step recovery diode can generate high-frequency signals with high efficiency, up to 50%, which means that it can minimize the power loss and heat dissipation in the circuit. A step recovery diode also has a low power consumption, which makes it suitable for battery-powered applications.
However, a step recovery diode has some limitations, such as its sensitivity to temperature and bias voltage, which can affect its performance and stability. A step recovery diode also has a limited output power, which means that it may require amplification or modulation for some applications.
In summary, a step recovery diode is a powerful and versatile semiconductor device that offers fast switching time, high frequency response, and low distortion. Its unique properties make it a valuable component in many applications that require pulse generation and shaping, especially in the field of radar systems, pulse generators, and high-speed communication.
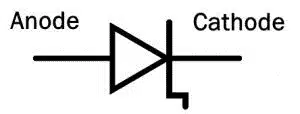
Peltier or Thermal Diode
A Peltier or thermal diode is a type of semiconductor device that is designed to generate or absorb heat when a current flows through it, based on the Peltier effect. It is also known as a thermoelectric diode or a heat pump diode. The Peltier or thermal diode was first discovered by Jean Charles Athanase Peltier, a French physicist, in 1834.
A Peltier or thermal diode is made of two different types of semiconductor materials, such as n-type and p-type materials, that are connected in series. When a current flows through the diode, the electrons in the n-type material move from the hot side to the cold side, while the holes in the p-type material move from the cold side to the hot side, which creates a temperature difference across the diode. This temperature difference can be used to generate or absorb heat, depending on the direction of the current flow.
The main advantage of a Peltier or thermal diode over other types of heat pumps is its simplicity, compactness, and versatility. A Peltier or thermal diode does not require any moving parts or refrigerants to generate or absorb heat, which makes it suitable for applications that require low-power and low-maintenance solutions, such as cooling or heating of electronic components and sensors. A Peltier or thermal diode is also small and lightweight, which makes it suitable for portable and space-constrained applications.
Another advantage of a Peltier or thermal diode is its high efficiency and low noise. A Peltier or thermal diode can generate or absorb heat with high efficiency, up to 50%, which means that it can minimize the power loss and heat dissipation in the circuit. A Peltier or thermal diode also has a low noise and vibration, which means that it can provide high-quality cooling or heating without introducing unwanted noise or disturbance.
However, a Peltier or thermal diode has some limitations, such as its sensitivity to temperature and current, which can affect its performance and stability. A Peltier or thermal diode also has a limited temperature range, which means that it may require additional cooling or heating devices for high-temperature or low-temperature applications.
In summary, a Peltier or thermal diode is a powerful and versatile semiconductor device that offers heat generation or absorption, simplicity, and high efficiency. Its unique properties make it a valuable component in many applications that require cooling or heating of electronic components and sensors, especially in the field of thermal management and energy harvesting.
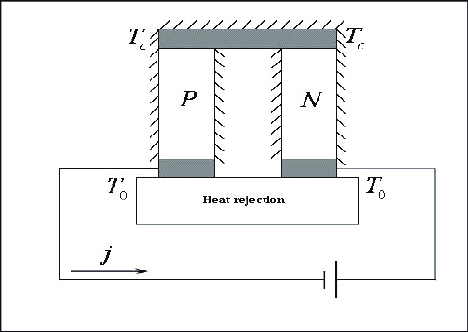
Vacuum Diode
A vacuum diode is a type of electronic device that consists of a vacuum-sealed glass envelope containing two electrodes, a cathode and an anode, that are separated by a vacuum gap. It is also known as a thermionic diode or a Fleming valve. The vacuum diode was first invented by John Ambrose Fleming, a British engineer, in 1904.
A vacuum diode works on the principle of thermionic emission, where the cathode emits electrons when it is heated to a high temperature, and the anode attracts the electrons to create a current flow through the vacuum gap. The vacuum gap prevents the electrons from colliding with gas molecules, which allows the diode to operate at high voltages and frequencies.
The main advantage of a vacuum diode over other types of diodes is its high voltage handling capability and low noise. A vacuum diode can withstand high voltages, up to several kilovolts, without being damaged or destroyed, which makes it suitable for applications that require high-voltage rectification or detection, such as radio receivers and power supplies. A vacuum diode also has a low noise and distortion, which means that it can provide high-quality signals without introducing unwanted noise or distortion.
Another advantage of a vacuum diode is its high frequency response and low capacitance. A vacuum diode can respond very quickly to changes in voltage and current, with response times of nanoseconds or less, which makes it suitable for applications that require high-speed switching or detection, such as microwave detectors and mixers. A vacuum diode also has a low capacitance, which means that it can handle high-frequency signals without introducing unwanted capacitance or delay.
However, a vacuum diode has some limitations, such as its sensitivity to temperature and aging, which can affect its performance and stability. A vacuum diode also has a limited current range, which means that it may require additional current regulation or protection devices for high-current applications.
In summary, a vacuum diode is a powerful and versatile electronic device that offers high voltage handling capability, low noise, and high-frequency response. Its unique properties make it a valuable component in many applications that require high-voltage rectification or detection, especially in the field of radio communication and power electronics.
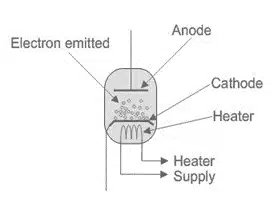
Conclusion
In conclusion, diodes are essential semiconductor devices that play a critical role in modern electronics. They are used in a wide range of applications, from rectification and detection to regulation and protection. In this blog post, we have explored various types of diodes, including the p-n junction diode, Schottky diode, Zener diode, PIN diode, Gunn diode, avalanche diode, backward diode, gold-doped diode, constant current diode, step recovery diode, Peltier or thermal diode, and vacuum diode. Each type of diode has its unique properties and advantages, which make it suitable for specific applications. By understanding the characteristics and capabilities of different types of diodes, engineers and designers can select the most appropriate diode for their specific needs and optimize the performance and efficiency of their electronic circuits.
FAQs about Types of Diodes
- What is a diode?
A diode is a two-terminal semiconductor device that allows current to flow in one direction but blocks it in the opposite direction; it is widely used in electronic circuits.
- What are the different types of diodes?
The different types of diodes include rectifying diodes, Zener diodes, light-emitting diodes (LEDs), and silicon-controlled rectifiers (SCRs).
- What are the applications of diodes?
Diodes are used in a wide range of applications, including power supply circuits, radio receivers, computers, and electric vehicles.
- What are the advantages and limitations of diodes?
The advantages of diodes include their ability to block current flow in one direction and their wide range of applications; the limitations of diodes include their temperature and voltage limitations and the presence of reverse current flow in certain diodes.
- How do I choose the right diode for my specific application?
When choosing the right diode for your specific application, consider factors such as operating voltage, current rating, and environmental conditions. It’s also important to consult the diode’s datasheet for more information on its specific characteristics.